Skip to main content
Circular particle colliders are the most important tool in modern high energy physics (HEP) for investigating at the largest mass and the smallest space scales. A key element of a circular accelerator is its magnet system. The magnet system is composed of large numbers of various types of magnets: dipoles and quadrupoles, to guide and steer the particle beams and high-order magnets used to focus the beams and increase the number of collisions and consequently the data gathered by the experiments.
FCC-ee
A high-luminosity lepton circular collider like FCC-ee, will have more than 9,000 magnets which steer and focus the two beams and deliver the required luminosities at the collision points where the experiments will be located. FCC-ee consists of the main dipoles, bending the trajectories of the particles in the plane of the machine, and quadrupole magnets that focus the particles towards the central orbit all along the 100 km circumference. In addition, numerous higher-order magnets (i.e. sextupoles, octopoles, etc.) will be used for focusing the beams to the interaction point.
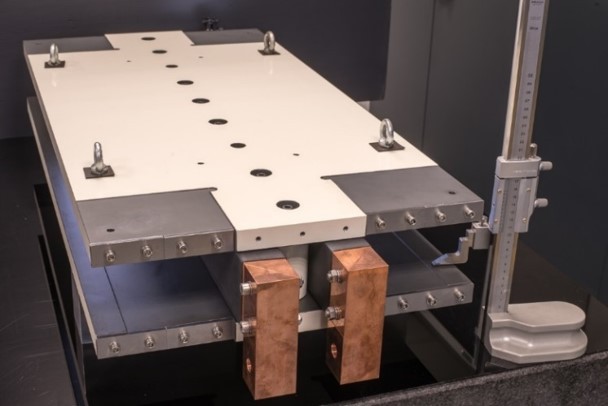
Top: First prototype of a dipole magnet for a future lepton collider (FCC-ee). Bottom: FCC-ee quadrupole test magnet installed on the magnetic measurement bench at CERN. (Image Credits: CERN)
The philosophy is to design the simplest (in terms of magnetic elements) high performance system, using state-of-the-art techniques whenever possible. A major innovation for FCC-ee, compared to LEP, is the possible implementation of a dual-aperture approach which would halve the total number of main magnets and could also save 50% in electric power consumtion.
FCC-hh high-field magnets
Producing high-field superconducting magnets is one of the enabling technologies for energy frontier hadron colliders like the proposed FCC-hh. Magnets guide the particle beams on a quasi circular track for billions of turns: the higher the energy of the circulating particles, the stronger the magnetic field must be. To steer the rigid 50 TeV beams on a 100 km circumference track, the bending magnets must produce a field of 16 Tesla, twice as strong as that produced by the LHC dipole magnets. This requires entirely new design concepts, novel materials, cost optimised production and operation approaches.
Researchers in the US have demonstrated an advanced accelerator dipole magnet with a field of 14.1 T – the highest ever achieved for such a device at an operational temperature of 4.5 K. This milestone is the work of the US Magnet Development Program (MDP), which includes Fermilab, Lawrence Berkeley National Laboratory (LBNL), the National High-Field Magnetic Field Laboratory and Brookhaven National Laboratory.
The FCC magnet R&D programme responds to this challenge, with two main goals: to extend the range of operation of accelerator magnets based on Low Temperature Superconductors (LTS) up to 16 Tesla, and to explore the technological challenges inherent in the use of High Temperature Superconductors (HTS) for accelerator magnets in the 20 Tesla range. This is translated into a staged approach covering both LTS and HTS technologies. Nb3Sn LTS magnets designed to attain 16 Tesla are being studied, assisted by a material and magnet technology programme. At the same time, a simple and modular design of a 5 Tesla HTS insert is being pursued, to approach the 20 Tesla barrier.
Key challenges
-
Produce a conceptual and engineering design a 16 Tesla accelerator dipole magnet in LTS
-
Produce a conceptual design and proof-of-principle of a 20 Tesla accelerator dipole magnet
-
Achieve accelerator-grade field quality in a 50 mm aperture
-
Explore different coil geometries and novel magnet concepts
-
Master large scale manufacturing aspects
-
Optimise manufacturing and operation costs